Back from their strenuous vacation studying female bicyclists in Paris, Sherlock Holmes and Dr. Watson have returned to their favourite chairs in front of the fireplace.
Holmes puffs on his pipe before turning towards Dr. Watson: What can you tell me about mid-ocean ridges, Watson?
Dr. Watson, being rather perplexed by the unexpected choice of subject:
– Well, I suppose that we may assume that the ridges in question are located in the middle of the ocean, or thereabouts.
Holmes, perhaps having expected a more scientific answer, erupts: Is that the best you can do!?
Dr. Watson realised that his first chance of demonstrating brilliance had failed.
– Well, I of course know that the ridges in question are volcanic in nature and look like a mountain chain along the seabed. Because they are produced by upwelling magma that has to go somewhere after reaching the seabed, the ridges are continuously producing new oceanic crust. How about that for an answer, Holmes?
Holmes replies: A slight improvement, Watson; however, you seem to have missed THE crucial point …
– THE crucial point, Holmes, what might that be, Dr. Watson wonders.
Holmes: You forgot to explain the MID-OCEAN aspect.
– The mid-ocean aspect, Holmes – what more is there to say about that?
Holmes: How about explaining what it means and what it implies?
– I guess it means that the spreading ridge is creating the new oceanic crust at roughly the same distance from two pieces of land on each side of it. In addition, it probably means that they used to be one piece of land before it split into two pieces of land, Dr. Watson explains. This is part of what plate tectonics is all about, he adds.
You are getting closer, Watson, but not as close as I had expected from a man of your class and education, Holmes states to the great disappointment of Dr. Watson, I guess I must provide the answer myself.
Holmes continues: You see Watson, for the mid-ocean ridge to stay mid-ocean, the pieces of land on each side of it will have to move at an equal rate opposite to each other – and that begs the question, what might make the two pieces of land move at nearly equal speed in opposite directions?
– That is very simple – now that I understand where you are heading, Holmes, Dr. Watson continues with what he regards as a climb to restore his honour again
– I have heard that subduction of plates is providing ca. 80 % of the force that makes plates move, and the remaining ca. 20 % is provided by what is called ridge push [1,2]. In other words, subduction zones pull and ridges push each piece of land in equal amounts to make the plates move at equal speed and make the ridge continue to stay in the middle.
Holmes comments: OK, Watson, I can hear that you have spent a lot of time reading books and articles and less time thinking about what you read.
There might be another explanation, Watson, but I am not saying that it is the correct explanation. What if one piece of land is stagnant and the mid-ocean ridge itself is moving away from it, while a subducting segment simultaneously moves the other piece of land in just the right amount to keep the ridge in the middle?
– That sounds like a crazy idea, Holmes, I hope that you are joking, Dr. Watson exclaims.
Holmes smiles: Ok then, let us look at a present case on Earth and then do some thinking. Everybody agrees that Africa and South America are moving away from each other, and sure enough, the mid-ocean ridge is found roughly in the middle between the two. However, Watson, where are the subduction zones fitting your explanation?
Dr. Watson explains that there is indeed a subduction zone connected to South America.
Holmes asks cunningly: Where is this subduction zone located?
– It runs along the entire west coast of South America and continues even further northwards, Dr. Watson explains.
Holmes: OK, Watson, there is a subduction zone along the South American continent. However, it cannot be pulling South America westwards – on the contrary, it is pulling the Pacific Sea bottom eastwards. For the subduction model to be correct about this mid-ocean ridge formation, something would have to be pulling the oceanic crust between the mid-ocean ridge and South America westwards.
Something is moving South America westwards relative to Africa, and it does not look like it is related to subduction. Where is the subduction zone that is pulling Africa away from the mid-ocean ridge?
My dear friend Watson, do you think that the pull from the subduction zone along western South America is pulling the Pacific oceanic crust, the Indian Ocean oceanic crust, and subsequently Africa, away from South America?
Without expecting an answer, Holmes continues; If two independent forces pulled the two plates away from each other, the supposedly dominating (80 %) and independent forces acting on each plate would have to make the plates move at an equal rate to keep the mid-ocean ridge mid-ocean. How likely is that?
If South America is just sitting there while Africa is pulled away due to the strange actions of the South American subduction, what makes the mid-ocean ridge move along with Africa in just the right amount to keep it mid-ocean?
Dr. Watson remains silent while Holmes continues lecturing: By sheer coincidence, South America and Africa might just happen to be pulled away from each other in precisely the right amount relative to the mid-ocean ridge due to unknown forces. Alternatively, the mid-ocean ridge itself might be pushing the two continents away from itself by an equal amount on each side. This would be true if the upwards flow of mass beneath the ridge is deviated laterally and equally to each side. Although this would keep the ridge mid-ocean, it would seriously expand the assumed role of ridge push.
In other words, the 80 % pull dominance is hard to explain in our example, as it would require a coordinated and fine-tuned pull acting on the two plates from subduction zones, or a drifting mid-ocean ridge to satisfy observations. Moreover, as stated earlier, the dominant role of ridge push goes against what we have been told.
Holmes asks: We are stuck, Watson – or what are your thoughts?
Dr. Watson remains silent for a while and then suggests:
– What if we are missing something? There must be some more information out there that we might utilise.
Holmes: Exactly, Watson, to get closer to an explanation, we have to look at the coastal regions of the two separating landmasses. On the west coast of Africa, which used to be attached to South America, we observe that the crust has been faulted and stretched westwards. Millions of years ago, many continental fault blocks moved away from the crustal plate of Africa out to the first appearing oceanic crust in the emerging rift [3,4,5] – around the location where the mid-ocean ridge was once born. A similar situation can be observed along the coast of eastern South America.
– But, but, Dr. Watson injects, how is this fault pattern possible if the emerging mid-ocean ridge is pushing each side of the rift away from it? Why would the fault blocks be moving towards – and not away from a pushing and expanding oceanic crust? That does not make sense, Holmes!
Holmes exclaims: Ah, finally, some proof that your grey cells are working, Watson!
Holmes continues: This shows us that IF the initial ridge push was moving the African and South American plates away from each other, the push must have been applied beneath the crustal level where the fault blocks are being observed today. As you have discovered, the observed fault pattern testifies against push acting on the upper crust. However, because we know that the continent rifted into two parts that drifted apart at the same pace from the initial rift, some ridge push must have been acting at deeper levels.
This begs a few simple questions: at what depth is the main force from the ridge push applied, what conditions would allow against-the-push extension of the upper crust, and what is the thickness of a moving continent?
– Excellent questions, Holmes – you probably would not ask unless you had this figured out, Dr. Watson adds.
Holmes continues without hesitation: Indeed, but before we dive into these mysteries, we have to go back to the theory explaining ridge push [6]. You see, Watson, the ridge push is usually estimated by calculating the gravitational forces pulling the tall ridge down and then pushing the oceanic crust sideways. This, however, might not tell the entire story. The young oceanic crust is not very thick, maybe a few km after cooling a bit. However, it acts as a rigid lid on top of the hot, upwelling asthenosphere. The height of the ridge is therefore part of a force balance between downwards acting forces and upwards acting forces.
The upwelling asthenosphere is hotter and therefore less dense than its surroundings. When approaching the seabed, any volatiles within the rising masses will expand and lower the density even more. The lower density makes the hot asthenosphere buoyant relative to its surroundings. This density difference, therefore, produces static buoyancy forces in addition to the dynamic push due to its upward movement. The density contrast between the upwelling masses and surroundings is diminishing as you go deeper into the asthenosphere because down there, at a given depth, the temperature must be the same in the plume and surroundings.
The implication of this is that the forces creating the upwards movement of the asthenosphere are created in the upper parts of the plume, because it has ascended and thereby ended up being in thermal disequilibrium with its surroundings. This is a very interesting observation as it implies that something has to destabilize the asthenosphere first to get the upwelling process started before it becomes self-sustained by thermal/density instability. We will get back to that.
However, a large volume within the lower sections of the ridge plume is also set in motion due to frictional forces and the need to replace the volume of the upwelling masses. Because the deeper distal sections of the plume are in near thermal- and density-equilibrium with their surroundings, they are not that buoyant and therefore deviate laterally at depth without producing significant ridge uplift.
In conclusion, the height of the mid-ocean ridge might tell a relevant story about the pressure generated by the buoyant masses (the ridge push), however, it might tell an incomplete story about the amount of mass being moved laterally at depth. Consequently, the lateral velocity of the asthenosphere might also be a lot faster than indicated by the oceanic crust production and maybe even larger than the velocity of pushed continents [7, 8].
Holmes continues: Remember also that forces acting on the upper, thin oceanic crust cannot be responsible for pushing continents apart. This is particularly true during the early phase of rifting, where an oceanic crust has yet to form. The seaward extension of rifted continents confirms that no ridge-push forces have been acting on the upper crust in the rift direction.
The observation of mid-ocean ridges and this upper crust extension leaves us with the only possibility that, specifically, the deeper layers of the upwelling and deflected mantle flow must be responsible for the plate-moving forces usually assigned to ridge push. These forces might also contribute by more than 20 %, as our Africa-South America example illustrates.
Let us look at a figure, Watson.
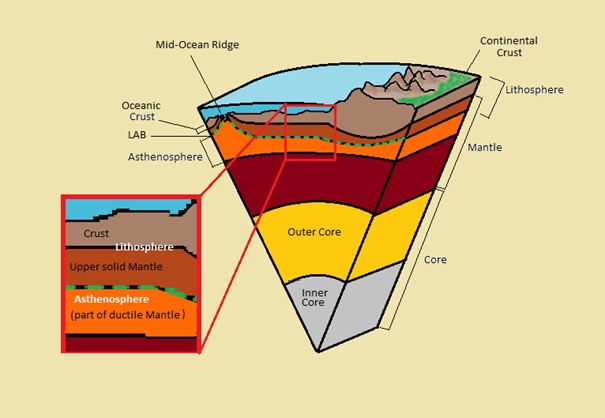
In this figure, we can clearly see that the upper boundary of the asthenosphere is formed by the thickness and weight of the lithosphere above it. Because the lower boundary maintains a spherical form, the implication is that beneath thick continents, the asthenosphere is much thinner than beneath fresh oceanic crust. Hence, when a continent is rifting due to the upwelling asthenosphere, the channel available for lateral flow beneath the lithosphere is limited. The relative velocity between the broken lithospheric parts and the flowing asthenosphere is therefore large for thick lithospheres.
It is somewhat unexpected that the thickest and strongest lithospheres will experience the largest frictional forces from the flowing asthenosphere. However, the high relative velocity between asthenosphere and lithosphere is not sustained for very long (in geologic terms). After some time, the rifted plates have accelerated to reach some terminal velocity where frictional forces and movement of plates/masses become balanced.
Holmes continues: We may now answer the question about the thickness of moving plates and where the ridge push force is applied. The push force is applied by the asthenosphere in the boundary layer within itself beneath the lithosphere. The moving parts include the entire lithosphere as well as the asthenosphere, where the velocity varies with depth according to the velocity profile within itself [8, 9].
We will later deal with the against-the-push extension during rifting. First, we must discuss what ultimately happens to the moving asthenosphere.
The asthenosphere must go somewhere after being set in motion, and this somewhere simply must be the subduction zones. Therefore, the frictional forces acting between the moving asthenosphere and the lithospheric plates above must also act on the subducting slabs. This would add to the pull from the thin but dense subducting slabs.
Thus, even if the South American subduction zone is moving in the “wrong” direction, it might be accompanied by asthenosphere flowing westwards beneath South America and bending sharply downwards as it encounters the upper surface of the subducting slabs. If at this point, the asthenosphere has become slightly colder than during its upwelling period, it might even add some extra negative buoyancy and (slab) pull away from the mid-ocean ridge.
The same might be the case for the asthenosphere beneath the subducting slabs diving beneath South America from the west. It may seem that ridge push and slab pull must be coordinated in some way, even over long distances.
– Indeed, Holmes, Dr. Watson manages to inject before Holmes continues:
Continents might indeed be set in motion by dominating movements in the asthenosphere originating at spreading ridges, but it does not explain hyperextension and movement of broken crustal blocks towards the direction of a push, even if the push occurs at deeper levels. This seems like a serious flaw and a contradiction within our beautiful theory. However, the explanation might be as simple as it is complicated.
The other contradiction we have unravelled is the tendency for an initially thick lithosphere to be more easily torn apart by a moving asthenosphere than a thin one. This also requires some more thinking.
How about a nightcap, Watson?
– I was hoping you would not say that – or, in a way, I was hoping you would say that … You know what I mean, Holmes, Dr. Watson manages to say.
Holmes: OK, then, we will continue tomorrow. We should not exhaust ourselves on the first day after returning from a hard vacation.
Cheers, Watson!
HANS K JOHNSEN
Inspired by Arthur Conan Doyle
References
- Schellart, W. P., 2004, Quantifying the net slab pull force as a driving mechanism for plate tectonics, Geophysical Research Letters, Vol. 31, L07611, doi:10.1029/2004GL019528
- Forsyth, D. W., Uyeda, S., 1975, On the relative importance of the driving forces of plate motion, Geophys. J. R. Astron. Soc., 43,163 – 200.
- Buiter S., J., H., Torsvik T., H., 2014, A review of Wilson Cycle plate margins: A role for mantle plumes in continental break-up along sutures?, Gondwana research, 26, 627-653, http://dx.doi.org/10.1016/j.gr.2014.02.007
- Pinto, V.H.G.; Manatschal, G.; Karpoff, A.M.; Masini, E.; Victor, R.A.; Viana, A.R.; Ulrich, M., 2023, Mass-Transfer and Fluid Flow along Extensional Detachment Faults in Hyperextended Rift Systems: The Examples of Tasna in the Alps, Mauléon in the Pyrenees, and Hobby High Offshore Iberia. Geosciences,13, 374. https://doi.org/10.3390/geosciences13120374
- Pichel, L. M., Huismans, R. S., Gawthorpe, R., Faleide, J. I., Theunissen, T., 2022, Coupling crustal-scale rift architecture with passive margin salt tectonics: A geodynamic modeling approach. Journal of Geophysical Research: Solid Earth, 127, https://doi.org/10.1029/2022JB025177
- Lithgow-Bertelloni, C., 2014. Driving Forces: Slab Pull, Ridge Push. In: Harff, J., Meschede, M., Petersen, S., Thiede, J. (eds) Encyclopedia of Marine Geosciences. Springer, Dordrecht. https://doi.org/10.1007/978-94-007-6644-0_105-1
- Stotz, I. L., Iaffaldano, G., Davies, D. R., 2018, Pressure-driven Poiseuille flow: A major component of the torque-balance governing Pacific Plate motion. Geophysical Research Letters, 45, 117–125, https://doi.org/10.1002/2017GL075697
- Colli, L., Stotz,I.,L., Bunge, H.-P., Smethurst, Clark, S., Iaffaldano, G.,Tassara, A., Guillocheau,,F.,. Bianchi, M. C., 2014, Rapid South Atlantic spreading changes and coeval vertical motion in surrounding continents: Evidence for temporal changes of pressure-driven upper mantle flow, Tectonics, 32, 1304–1321, doi:10.1002/2014TC003612
- Richards, M. A., Lenardic, A., 2018. The Cathles parameter (Ct): A geodynamic definition of the asthenosphere and implications for the nature of plate tectonics. Geochemistry, Geophysics, Geosystems, 19, 4858–4875, https://doi.org/10.1029/2018GC007664